Default mode network
In neuroscience, the default mode network (DMN), also default network, or default state network, is a large-scale brain network primarily composed of the medial prefrontal cortex, posterior cingulate cortex/precuneus and angular gyrus. It is best known for being active when a person is not focused on the outside world and the brain is at wakeful rest, such as during daydreaming and mind-wandering. It can also be active during detailed thoughts related to external task performance.[3] Other times that the DMN is active include when the individual is thinking about others, thinking about themselves, remembering the past, and planning for the future.[4][5]
Default mode network | |
---|---|
![]() fMRI scan showing regions of the default mode network; the medial prefrontal cortex, the posterior cingulate cortex/precuneus and the angular gyrus | |
Anatomical terminology |
Though the DMN was originally noticed to be deactivated in certain goal-oriented tasks and is sometimes referred to as the task-negative network,[6] it can be active in other goal-oriented tasks such as social working memory or autobiographical tasks.[7] The DMN has been shown to be negatively correlated with other networks in the brain such as attention networks.[8]
Evidence has pointed to disruptions in the DMN of people with Alzheimer's and autism spectrum disorder.[4]
History
Hans Berger, the inventor of the electroencephalogram, was the first to propose the idea that the brain is constantly busy. In a series of papers published in 1929 he showed that the electrical oscillations detected by his device do not cease even when the subject is at rest. However, his ideas were not taken seriously, and a general perception formed among neurologists that only when a focused activity is performed does the brain (or a part of the brain) become active.[9]
But in the 1950s, Louis Sokoloff and his colleagues noticed that metabolism in the brain stayed the same when a person went from a resting state to performing effortful math problems suggesting active metabolism in the brain must also be happening during rest.[4] In the 1970s, David H. Ingvar and colleagues observed blood flow in the front part of the brain became the highest when a person is at rest.[4] Around the same time, intrinsic oscillatory behavior in vertebrate neurons was observed in cerebellar Purkinje cells, inferior olivary nucleus and thalamus.[10]
In the 1990s, with the advent of positron emission tomography (PET) scans, researchers began to notice that when a person is involved in perception, language, and attention tasks, the same brain areas become less active compared to passive rest, and labeled these areas as becoming "deactivated".[4]
In 1995, Bharat Biswal, a graduate student at the Medical College of Wisconsin in Milwaukee, discovered that the human sensorimotor system displayed "resting-state connectivity," exhibiting synchronicity in functional magnetic resonance imaging (fMRI) scans while not engaged in any task.[11][12]
Later, experiments by neurologist Marcus E. Raichle's lab at Washington University School of Medicine and other groups [13] showed that the brain's energy consumption is increased by less than 5% of its baseline energy consumption while performing a focused mental task. These experiments showed that the brain is constantly active with a high level of activity even when the person is not engaged in focused mental work. Research thereafter focused on finding the regions responsible for this constant background activity level.[9]
Raichle coined the term "default mode" in 2001 to describe resting state brain function;[14] the concept rapidly became a central theme in neuroscience.[15] Around this time the idea was developed that this network of brain areas is involved in internally directed thoughts and is suspended during specific goal-directed behaviors. In 2003, Greicius and colleagues examined resting state fMRI scans and looked at how correlated different sections in the brain are to each other. Their correlation maps highlighted the same areas already identified by the other researchers.[16] This was important because it demonstrated a convergence of methods all leading to the same areas being involved in the DMN. Since then other resting state networks (RSNs) have been found, such as visual, auditory, and attention networks. Some of them are often anti-correlated with the default mode network.[8]

In the beginning to mid 2000s, researchers labeled the default mode network as the task negative network[6] because it was deactivated when participants had to perform tasks. DMN was thought to only be active during passive rest and then turned off during externally focused goal-directed tasks. However, studies have demonstrated the DMN to be active in external goal-directed tasks which are known to involve the DMN such as social working memory or autobiographical tasks.[7] The DMN also shows higher activation when behavioral responses are stable, and this activation is independent of self-reported mind wandering.[17]
Around 2007, the number of papers referencing the default mode network skyrocketed.[18] In all years prior to 2007, there were 12 papers published that referenced "default mode network" or "default network" in the title; however, between 2007 and 2014 the number increased to 1,384 papers. One reason for the increase in papers is a result of the robust effect of finding the DMN with resting state scans and independent component analysis (ICA).[13][19] Another reason is the DMN can be measured with short and effortless resting state scans, meaning they can be performed on any population including young children, clinical populations, and nonhuman primates.[4] A third reason is that the role of the DMN has been expanded to more than just a passive brain network.
Function
The default mode network is thought to be involved in several different functions:
It is potentially the neurological basis for the self:[18]
- Autobiographical information: Memories of collection of events and facts about one's self
- Self-reference: Referring to traits and descriptions of one's self
- Emotion of one's self: Reflecting about one's own emotional state
Thinking about others:[18]
- Theory of mind: Thinking about the thoughts of others and what they might or might not know
- Emotions of other: Understanding the emotions of other people and empathizing with their feelings
- Moral reasoning: Determining just and unjust result of an action
- Social evaluations: Good-bad attitude judgments about social concepts
- Social categories: Reflecting on important social characteristics and status of a group
- Social isolation: A perceived lack of social interaction[20].
Remembering the past and thinking about the future:[18]
- Remembering the past: Recalling events that happened in the past
- Imagining the future: Envisioning events that might happen in the future
- Episodic memory: Detailed memory related to specific events in time
- Story comprehension: Understanding and remembering a narrative
The default mode network is active during passive rest and mind-wandering[4] which usually involves thinking about others, thinking about one's self, remembering the past, and envisioning the future rather than the task being performed.[18] Recent work, however, has challenged a specific mapping between the default mode network and mind-wandering, given that the system is important in maintaining detailed representations of task information during working memory encoding.[21] Electrocorticography studies (which involve placing electrodes on the surface of a subject's scalp) have shown the default mode network becomes activated within a fraction of a second after participants finish a task.[22] Additionally, during attention demanding tasks, sufficient deactivation of the default mode network at the time of memory encoding has been shown to result in more successful long-term memory consolidation.[23]
Studies have shown that when people watch a movie,[24] listen to a story,[25][26] or read a story,[27] their DMNs are highly correlated with each other. DMNs are not correlated if the stories are scrambled or are in a language the person does not understand, suggesting that the network is highly involved in the comprehension and the subsequent memory formation of that story.[26] The DMN is shown to even be correlated if the same story is presented to different people in different languages,[28] further suggesting the DMN is truly involved in the comprehension aspect of the story and not the auditory or language aspect.
The default mode network has shown to deactivate during external goal-oriented tasks such as visual attention or cognitive working memory tasks, thus leading some researchers to label the network as the task-negative network.[6] However, when the tasks are external goal-oriented tasks that are known to be a role of the DMN, such as social working memory or an autobiographical task, the DMN is positively activated with the task and correlates with other networks such as the network involved in executive function.[7]
A hitherto unsuspected possibility is that the default network is activated by the immobilization inherent in the testing procedure (the patient is strapped supine on a stretcher and inserted by a narrow tunnel into a massive metallic structure). This procedure creates a sense of entrapment and, not surprisingly, the most commonly reported side-effect is claustrophobia. This alternative view is suggested by a recent article that links theory of mind to immobilization.[29]
Recent research has shown that the DMN is related to the perception of beauty, in which the network becomes activated in a generalized way to aesthetically moving domains such as artworks, landscapes and architecture. This would explain a deep inner feeling of pleasure related to aesthetics, interconnected with the sense of personal identity, due to the network functions related to the self.[30]
Anatomy
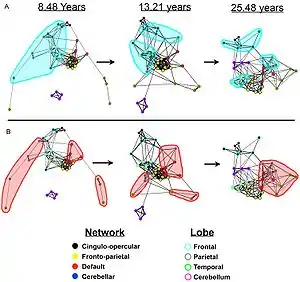
The default mode network is an interconnected and anatomically defined[4] set of brain regions. The network can be separated into hubs and subsections:
Functional hubs:[32] Information regarding the self
- Posterior cingulate cortex (PCC) & precuneus: Combines bottom-up (not controlled) attention with information from memory and perception. The ventral (lower) part of PCC activates in all tasks which involve the DMN including those related to the self, related to others, remembering the past, thinking about future, and processing concepts plus spatial navigation. The dorsal (upper) part of PCC involves involuntary awareness and arousal. The precuneus is involved in visual, sensorimotor, and attentional information.
- Medial prefrontal cortex (mPFC): Decisions about self processing such as personal information, autobiographical memories, future goals and events, and decision making regarding those personally very close such as family. The ventral (lower) part is involved in positive emotional information and internally valued reward.
- Angular gyrus: Connects perception, attention, spatial cognition, and action and helps with parts of recall of episodic memories
Dorsal medial subsystem:[32] Thinking about others
- Functional hubs: PCC, mPFC, and angular gyrus
- Dorsal medial prefrontal cortex (dmPFC): Involved in social directed thought such as determining or inferring the purpose of others' actions
- Temporoparietal junction (TPJ): Reflects on beliefs about others, also known as theory of mind
- Lateral temporal cortex: Retrieval of social semantic and conceptual knowledge
- Anterior temporal pole: Abstract conceptual information particularly social in nature
Medial temporal subsystem:[32] Autobiographical memory and future simulations
- Functional hubs: PCC, mPFC, and angular gyrus
- Hippocampus (HF+): Formation of new memories as well as remembering the past and imagining the future
- Parahippocampus (PHC): Spatial and scene recognition and simulation
- Retrosplenial cortex (RSC): Spatial navigation
- Posterior inferior parietal lobe (pIPL): Junction of auditory, visual, and somatosensory information and attention
The default mode network is most commonly defined with resting state data by putting a seed in the posterior cingulate cortex and examining which other brain areas most correlate with this area.[16] The DMN can also be defined by the areas deactivated during external directed tasks compared to rest.[14] Independent component analysis (ICA) robustly finds the DMN for individuals and across groups, and has become the standard tool for mapping the default network.[13][19]
It has been shown that the default mode network exhibits the highest overlap in its structural and functional connectivity, which suggests that the structural architecture of the brain may be built in such a way that this particular network is activated by default.[1] Recent evidence from a population brain-imaging study of 10,000 UK Biobank participants further suggests that each DMN node can be decomposed into subregions with complementary structural and functional properties. It has been a widespread practice in DMN research to treat its constituent nodes to be functionally homogeneous, but the distinction between subnodes within each major DMN node has mostly been neglected. However, the close proximity of subnodes which propagate hippocampal space-time outputs and subnodes that describe the global network architecture may enable default functions, such as autobiographical recall or internally-orientated thinking.[33]
In the infant brain, there is limited evidence of the default network, but default network connectivity is more consistent in children aged 9–12 years, suggesting that the default network undergoes developmental change.[8]
Functional connectivity analysis in monkeys shows a similar network of regions to the default mode network seen in humans.[4] The PCC is also a key hub in monkeys; however, the mPFC is smaller and less well connected to other brain regions, largely because human's mPFC is much larger and well developed.[4]
Diffusion MRI imaging shows white matter tracts connecting different areas of the DMN together.[18] The structural connections found from diffusion MRI imaging and the functional correlations from resting state fMRI show the highest level of overlap and agreement within the DMN areas.[1] This provides evidence that neurons in the DMN regions are linked to each other through large tracts of axons and this causes activity in these areas to be correlated with one another.
Pathophysiology
The default mode network has been hypothesized to be relevant to disorders including Alzheimer's disease, autism, schizophrenia, depression, chronic pain, posttraumatic stress disorder and others.[4][34] In particular, the DMN has also been reported to show overlapping yet distinct neural activity patterns across different mental health conditions, such as when directly comparing attention deficit hyperactivity disorder and autism.[35]
People with Alzheimer's disease show a reduction in glucose (energy use) within the areas of the default mode network.[4] These reductions start off as slight decreases in mild patients and continue to large reductions in severe patients. Surprisingly, disruptions in the DMN begin even before individuals show signs of Alzheimer's disease.[4] Plots of the peptide amyloid-beta, which is thought to cause Alzheimer's disease, show the buildup of the peptide is within the DMN.[4] This prompted Randy Buckner and colleagues to propose the high metabolic rate from continuous activation of DMN causes more amyloid-beta peptide to accumulate in these DMN areas.[4] These amyloid-beta peptides disrupt the DMN and because the DMN is heavily involved in memory formation and retrieval, this disruption leads to the symptoms of Alzheimer's disease.
DMN is thought to be disrupted in individuals with autism spectrum disorder.[4][36] These individuals are impaired in social interaction and communication which are tasks central to this network. Studies have shown worse connections between areas of the DMN in individuals with autism, especially between the mPFC (involved in thinking about the self and others) and the PCC (the central core of the DMN).[37][38] The more severe the autism, the less connected these areas are to each other.[37][38] It is not clear if this is a cause or a result of autism, or if a third factor is causing both (confounding).
Lower connectivity between brain regions was found across the default network in people who have experienced long term trauma, such as childhood abuse or neglect, and is associated with dysfunctional attachment patterns. Among people experiencing posttraumatic stress disorder, lower activation was found in the posterior cingulate gyrus compared to controls, and severe PTSD was characterized by lower connectivity within the DMN.[34][39] Hyperconnectivity of the default network has been linked to rumination in first-episode depression[40] and chronic pain.[41] If the default mode network is altered, this can change the way one perceives events and their social and moral reasoning, thus making a person more susceptible to major depressive-like symptoms.[42]
Multivariate analysis reveals genetic associations of the resting DMN in psychotic bipolar disorder and schizophrenia.[43]
Modulation
The default mode network (DMN) may be modulated by the following interventions and processes:
- Acupuncture – Deactivation of the limbic brain areas and the DMN.[44] It has been suggested that this is due to the pain response.[45]
- Meditation – Structural changes in areas of the DMN such as the temporoparietal junction, posterior cingulate cortex, and precuneus have been found in meditation practitioners.[46] There is reduced activation and reduced functional connectivity of the DMN in long-term practitioners.[46] Various forms of nondirective meditation, including Transcendental Meditation[47] and Acem Meditation,[48] have been found to activate the DMN.
- Sleeping and resting wakefulness
- Resting wakefulness – Functional connectivity between nodes of the DMN is strong.[49]
- Onset of sleep – Decrease in connectivity between the DMN and the task-positive network.[49]
- Stage N2 of NREM sleep – Decrease in connectivity between the posterior cingulate cortex and medial prefrontal cortex.[49]
- Stage N3 of NREM sleep – Further decrease in connectivity between the PCC and MPFC.[49]
- REM sleep – Possible increase in connectivity between nodes of the DMN.[49]
- Sleep deprivation – Functional connectivity between nodes of the DMN in their resting-state is usually strong, but sleep deprivation results in a decrease in connectivity within the DMN.[50] Recent studies suggest a decrease in connectivity between the DMN and the task-positive network as a result of sleep loss.[51]
- Psychedelic drugs – Reduced blood flow to the PCC and mPFC was observed under the administration of psilocybin. These two areas are considered to be the main nodes of the DMN.[52] One study on the effects of LSD demonstrated that the drug desynchronizes brain activity within the DMN; the activity of the brain regions that constitute the DMN becomes less correlated.[53]
- Deep brain stimulation – Alterations in brain activity with deep brain stimulation may be used to balance resting state networks.[54]
- Psychotherapy – In PTSD, the abnormalities in the default mode network normalize in individuals who respond to psychotherapy interventions.[55][56]
- Antidepressants – Abnormalities in DMN connectivity are reduced following treatment with antidepressant medications in PTSD.[56]
- Physical Activity and Exercise – Physical Activity, and more likely Aerobic Training, may alter the DMN. In addition, sports experts are showing networks differences, notably of the DMN.[57][58][59]
Criticism
Some have argued the brain areas in the default mode network only show up together because of the vascular coupling of large arteries and veins in the brain near these areas, not because these areas are actually functionally connected to each other. Support for this argument comes from studies that show changing in breathing alters oxygen levels in the blood which in turn affects DMN the most.[4] These studies however do not explain why the DMN can also be identified using PET scans by measuring glucose metabolism which is independent of vascular coupling[4] and in electrocorticography studies[60] measuring electrical activity on the surface of the brain, and in MEG by measuring magnetic fields associated with electrophysiological brain activity that bypasses the hemodynamic response.[61]
The idea of a "default network" is not universally accepted.[62] In 2007 the concept of the default mode was criticized as not being useful for understanding brain function, on the grounds that a simpler hypothesis is that a resting brain actually does more processing than a brain doing certain "demanding" tasks, and that there is no special significance to the intrinsic activity of the resting brain.[63]
See also
References
- Horn, Andreas; Ostwald, Dirk; Reisert, Marco; Blankenburg, Felix (2013). "The structural-functional connectome and the default mode network of the human brain". NeuroImage. 102: 142–151. doi:10.1016/j.neuroimage.2013.09.069. PMID 24099851.
- Garrity, A.; Pearlson, G. D.; McKiernan, K.; Lloyd, D.; Kiehl, K. A.; Calhoun, V. D. (2007). "Aberrant default mode functional connectivity in schizophrenia". Am. J. Psychiatry. 164 (3): 450–457. doi:10.1176/ajp.2007.164.3.450. PMID 17329470.
- Sormaz, Mladen; Murphy, Charlotte; Wang, Hao-Ting; Hymers, Mark; Karapanagiotidis, Theodoros; Poerio, Giulia; Margulies, Daniel S.; Jefferies, Elizabeth; Smallwood, Jonathan (2018). "Default mode network can support the level of detail in experience during active task states". Proceedings of the National Academy of Sciences. 115 (37): 9318–9323. doi:10.1073/pnas.1721259115. PMC 6140531. PMID 30150393.
- Buckner, R. L.; Andrews-Hanna, J. R.; Schacter, D. L. (2008). "The Brain's Default Network: Anatomy, Function, and Relevance to Disease". Annals of the New York Academy of Sciences. 1124 (1): 1–38. Bibcode:2008NYASA1124....1B. CiteSeerX 10.1.1.689.6903. doi:10.1196/annals.1440.011. PMID 18400922.
- Lieberman, Matthew (2 September 2016). Social. Broadway Books. p. 19. ISBN 978-0-307-88910-2.
- Fox, Michael D.; Snyder, Abraham Z.; Vincent, Justin L.; Corbetta, Maurizio; Van Essen, David C.; Raichle, Marcus E. (2005-07-05). "The human brain is intrinsically organized into dynamic, anticorrelated functional networks". Proceedings of the National Academy of Sciences of the United States of America. 102 (27): 9673–9678. Bibcode:2005PNAS..102.9673F. doi:10.1073/pnas.0504136102. ISSN 0027-8424. PMC 1157105. PMID 15976020.
- Spreng, R. Nathan (2012-01-01). "The fallacy of a "task-negative" network". Frontiers in Psychology. 3: 145. doi:10.3389/fpsyg.2012.00145. ISSN 1664-1078. PMC 3349953. PMID 22593750.
- Broyd, Samantha J.; Demanuele, Charmaine; Debener, Stefan; Helps, Suzannah K.; James, Christopher J.; Sonuga-Barke, Edmund J. S. (2009). "Default-mode brain dysfunction in mental disorders: A systematic review". Neuroscience & Biobehavioral Reviews. 33 (3): 279–96. doi:10.1016/j.neubiorev.2008.09.002. PMID 18824195.
- Raichle, Marcus (March 2010). "The Brain's Dark Energy". Scientific American. 302 (3): 44–49. Bibcode:2010SciAm.302c..44R. doi:10.1038/scientificamerican0310-44. PMID 20184182.
- Llinas, R. R. (2014). "Intrinsic electrical properties of mammalian neurons and CNS function: a historical perspective". Front Cell Neurosci. 8: 320. doi:10.3389/fncel.2014.00320. PMC 4219458. PMID 25408634.
- Biswal, B; Yetkin, F. Z.; Haughton, V. M.; Hyde, J. S. (1995). "Functional connectivity in the motor cortex of resting human brain using echoplanar MRI". Magn Reson Med. 34 (4): 537–541. doi:10.1002/mrm.1910340409. PMID 8524021. S2CID 775793.
- Shen, H. H. (2015). "Core Concepts: Resting State Connectivity". Proceedings of the National Academy of Sciences. 112 (46): 14115–14116. Bibcode:2015PNAS..11214115S. doi:10.1073/pnas.1518785112. PMC 4655520. PMID 26578753.
- Kiviniemi, Vesa J.; Kantola, Juha-Heikki; Jauhiainen, Jukka; Hyvärinen, Aapo; Tervonen, Osmo (2003). "Independent component analysis of nondeterministic fMRI signal sources". NeuroImage. 19 (2 Pt 1): 253–260. doi:10.1016/S1053-8119(03)00097-1. PMID 12814576.
- Raichle, M. E.; MacLeod, A. M.; Snyder, A. Z.; Powers, W. J.; Gusnard, D. A.; Shulman, G. L. (2001). "Inaugural Article: A default mode of brain function". Proceedings of the National Academy of Sciences. 98 (2): 676–82. Bibcode:2001PNAS...98..676R. doi:10.1073/pnas.98.2.676. PMC 14647. PMID 11209064.
- Raichle, Marcus E.; Snyder, Abraham Z. (2007). "A default mode of brain function: A brief history of an evolving idea". NeuroImage. 37 (4): 1083–90. doi:10.1016/j.neuroimage.2007.02.041. PMID 17719799.
- Greicius, Michael D.; Krasnow, Ben; Reiss, Allan L.; Menon, Vinod (2003-01-07). "Functional connectivity in the resting brain: a network analysis of the default mode hypothesis". Proceedings of the National Academy of Sciences of the United States of America. 100 (1): 253–258. Bibcode:2003PNAS..100..253G. doi:10.1073/pnas.0135058100. ISSN 0027-8424. PMC 140943. PMID 12506194.
- Kucyi, Aaron (2016). "Spontaneous default network activity reflects behavioral variability independent of mind-wandering". PNAS. 113 (48): 13899–13904. doi:10.1073/pnas.1611743113. PMID 27856733.
- Andrews-Hanna, Jessica R. (2012-06-01). "The brain's default network and its adaptive role in internal mentation". The Neuroscientist: A Review Journal Bringing Neurobiology, Neurology and Psychiatry. 18 (3): 251–270. doi:10.1177/1073858411403316. ISSN 1089-4098. PMC 3553600. PMID 21677128.
- De Luca, M; Beckmann, CF; De Stefano, N; Matthews, PM; Smith, SM (2006-02-15). "fMRI resting state networks define distinct modes of long-distance interactions in the human brain". NeuroImage. 29 (4): 1359–1367. doi:10.1016/j.neuroimage.2005.08.035. PMID 16260155.
- Spreng, R.N., Dimas, E., Mwilambwe-Tshilobo, L., Dagher, A., Koellinger, P., Nave, G., Ong, A., Kernbach, J.M., Wiecki, T.V., Ge, T., Holmes, A.J., Yeo, B.T.T., Turner, G.R., Dunbar, R.I.M., Bzdok, D (2020). "The default network of the human brain is associated with perceived social isolation". Nature Publishing Group. Cite journal requires
|journal=
(help)CS1 maint: multiple names: authors list (link) - Sormaz, Mladen; Murphy, Charlotte; Wang, Hao-ting; Hymers, Mark; Karapanagiotidis, Theodoros; Poerio, Giulia; Margulies, Daniel S.; Jefferies, Elizabeth; Smallwood, Jonathan (2018-08-24). "Default mode network can support the level of detail in experience during active task states". Proceedings of the National Academy of Sciences. 115 (37): 9318–9323. doi:10.1073/pnas.1721259115. ISSN 0027-8424. PMC 6140531. PMID 30150393.
- Dastjerdi, Mohammad; Foster, Brett L.; Nasrullah, Sharmin; Rauschecker, Andreas M.; Dougherty, Robert F.; Townsend, Jennifer D.; Chang, Catie; Greicius, Michael D.; Menon, Vinod (2011-02-15). "Differential electrophysiological response during rest, self-referential, and non-self-referential tasks in human posteromedial cortex". Proceedings of the National Academy of Sciences of the United States of America. 108 (7): 3023–3028. Bibcode:2011PNAS..108.3023D. doi:10.1073/pnas.1017098108. ISSN 1091-6490. PMC 3041085. PMID 21282630.
- Lefebvre, Etienne; D’Angiulli, Amedeo (2019). "Imagery-Mediated Verbal Learning Depends on Vividness–Familiarity Interactions: The Possible Role of Dualistic Resting State Network Activity Interference". Brain Sciences. 9 (6): 143. doi:10.3390/brainsci9060143. ISSN 2076-3425. PMC 6627679. PMID 31216699.
- Hasson, Uri; Furman, Orit; Clark, Dav; Dudai, Yadin; Davachi, Lila (2008-02-07). "Enhanced intersubject correlations during movie viewing correlate with successful episodic encoding". Neuron. 57 (3): 452–462. doi:10.1016/j.neuron.2007.12.009. ISSN 0896-6273. PMC 2789242. PMID 18255037.
- Lerner, Yulia; Honey, Christopher J.; Silbert, Lauren J.; Hasson, Uri (2011-02-23). "Topographic mapping of a hierarchy of temporal receptive windows using a narrated story". The Journal of Neuroscience. 31 (8): 2906–2915. doi:10.1523/JNEUROSCI.3684-10.2011. ISSN 1529-2401. PMC 3089381. PMID 21414912.
- Simony, Erez; Honey, Christopher J; Chen, Janice; Lositsky, Olga; Yeshurun, Yaara; Wiesel, Ami; Hasson, Uri (2016-07-18). "Dynamic reconfiguration of the default mode network during narrative comprehension". Nature Communications. 7 (1): 12141. Bibcode:2016NatCo...712141S. doi:10.1038/ncomms12141. ISSN 2041-1723. PMC 4960303. PMID 27424918.
- Regev, Mor; Honey, Christopher J.; Simony, Erez; Hasson, Uri (2013-10-02). "Selective and invariant neural responses to spoken and written narratives". The Journal of Neuroscience. 33 (40): 15978–15988. doi:10.1523/JNEUROSCI.1580-13.2013. ISSN 1529-2401. PMC 3787506. PMID 24089502.
- Honey, Christopher J.; Thompson, Christopher R.; Lerner, Yulia; Hasson, Uri (2012-10-31). "Not lost in translation: neural responses shared across languages". The Journal of Neuroscience. 32 (44): 15277–15283. doi:10.1523/JNEUROSCI.1800-12.2012. ISSN 1529-2401. PMC 3525075. PMID 23115166.
- Tsoukalas, Ioannis (2017). "Theory of Mind: Towards an Evolutionary Theory". Evolutionary Psychological Science. 4: 38–66. doi:10.1007/s40806-017-0112-x.Pdf.
- Starr, G. Gabrielle; Stahl, Jonathan L.; Belfi, Amy M.; Isik, Ayse Ilkay; Vessel, Edward A. (2019-09-04). "The default-mode network represents aesthetic appeal that generalizes across visual domains". Proceedings of the National Academy of Sciences. 116 (38): 19155–19164. doi:10.1073/pnas.1902650116. ISSN 0027-8424. PMC 6754616. PMID 31484756.
- Fair, Damien A.; Cohen, Alexander L.; Power, Jonathan D.; Dosenbach, Nico U. F.; Church, Jessica A.; Miezin, Francis M.; Schlaggar, Bradley L.; Petersen, Steven E. (2009). Sporns, Olaf (ed.). "Functional Brain Networks Develop from a 'Local to Distributed' Organization". PLOS Computational Biology. 5 (5): e1000381. Bibcode:2009PLSCB...5E0381F. doi:10.1371/journal.pcbi.1000381. PMC 2671306. PMID 19412534.
- Andrews-Hanna, Jessica R.; Smallwood, Jonathan; Spreng, R. Nathan (2014-05-01). "The default network and self-generated thought: component processes, dynamic control, and clinical relevance". Annals of the New York Academy of Sciences. 1316 (1): 29–52. Bibcode:2014NYASA1316...29A. doi:10.1111/nyas.12360. ISSN 1749-6632. PMC 4039623. PMID 24502540.
- Kernbach, J.M.; Yeo, B.T.T.; Smallwood, J.; Margulies, D.S.; Thiebaut; de Schotten, M.; Walter, H.; Sabuncu, M.R.; Holmes, A.J.; Gramfort, A.; Varoquaux, G.; Thirion, B.; Bzdok, D. (2018). "Subspecialization within default mode nodes characterized in 10,000 UK Biobank participants". Proc. Natl. Acad. Sci. 115 (48): 12295–12300. doi:10.1073/pnas.1804876115. PMC 6275484. PMID 30420501.
- Akiki, Teddy J.; Averill, Christopher L.; Wrocklage, Kristen M.; Scott, J. Cobb; Averill, Lynnette A.; Schweinsburg, Brian; Alexander-Bloch, Aaron; Martini, Brenda; Southwick, Steven M.; Krystal, John H.; Abdallah, Chadi G. (2018). "Default mode network abnormalities in posttraumatic stress disorder: A novel network-restricted topology approach". NeuroImage. 176: 489–498. doi:10.1016/j.neuroimage.2018.05.005. ISSN 1053-8119. PMC 5976548. PMID 29730491.
- Kernbach, Julius M.; Satterthwaite, Theodore D.; Bassett, Danielle S.; Smallwood, Jonathan; Margulies, Daniel; Krall, Sarah; Shaw, Philip; Varoquaux, Gaël; Thirion, Bertrand; Konrad, Kerstin; Bzdok, Danilo (17 July 2018). "Shared endo-phenotypes of default mode dysfunction in attention deficit/hyperactivity disorder and autism spectrum disorder". Translational Psychiatry. 8 (1): 133. doi:10.1038/s41398-018-0179-6. PMC 6050263. PMID 30018328.
- Vigneshwaran S, Mahanand B. S., Suresh S, Sundararajan N. Identifying differences in brain activities and an accurate detection of autism spectrum disorder using resting state functional-magnetic resonance imaging: A spatial filtering approach. Medical image analysis. 2017;35:375–389. doi:10.1016/j.media.2016.08.003.
- Washington, Stuart D.; Gordon, Evan M.; Brar, Jasmit; Warburton, Samantha; Sawyer, Alice T.; Wolfe, Amanda; Mease-Ference, Erin R.; Girton, Laura; Hailu, Ayichew (2014-04-01). "Dysmaturation of the default mode network in autism". Human Brain Mapping. 35 (4): 1284–1296. doi:10.1002/hbm.22252. ISSN 1097-0193. PMC 3651798. PMID 23334984.
- Yerys, Benjamin E.; Gordon, Evan M.; Abrams, Danielle N.; Satterthwaite, Theodore D.; Weinblatt, Rachel; Jankowski, Kathryn F.; Strang, John; Kenworthy, Lauren; Gaillard, William D. (2015-01-01). "Default mode network segregation and social deficits in autism spectrum disorder: Evidence from non-medicated children". NeuroImage: Clinical. 9: 223–232. doi:10.1016/j.nicl.2015.07.018. PMC 4573091. PMID 26484047.
- Dr. Ruth Lanius, Brain Mapping conference, London, November 2010
- Zhu, X; Wang, X; Xiao, J; Liao, J; Zhong, M; Wang, W; Yao, S (2012). "Evidence of a dissociation pattern in resting-state default mode network connectivity in first-episode, treatment-naive major depression patients". Biological Psychiatry. 71 (7): 611–7. doi:10.1016/j.biopsych.2011.10.035. PMID 22177602.
- Kucyi, A; Moayedi, M; Weissman-Fogel, I; Goldberg, M. B.; Freeman, B. V.; Tenenbaum, H. C.; Davis, K. D. (2014). "Enhanced medial prefrontal-default mode network functional connectivity in chronic pain and its association with pain rumination". Journal of Neuroscience. 34 (11): 3969–75. doi:10.1523/JNEUROSCI.5055-13.2014. PMC 6705280. PMID 24623774.
- Sambataro, Fabio; Wolf, Nadine; Giusti, Pietro; Vasic, Nenad; Wolf, Robert (October 2013). "Default mode network in depression: A pathway to impaired affective cognition?" (PDF). Clinical Neuralpyschiatry. 10: 212–216. Archived from the original (PDF) on 29 August 2017. Retrieved 28 September 2017.
- Meda, Shashwath A.; Ruaño, Gualberto; Windemuth, Andreas; O’Neil, Kasey; Berwise, Clifton; Dunn, Sabra M.; Boccaccio, Leah E.; Narayanan, Balaji; Kocherla, Mohan (2014-05-13). "Multivariate analysis reveals genetic associations of the resting default mode network in psychotic bipolar disorder and schizophrenia". Proceedings of the National Academy of Sciences. 111 (19): E2066–E2075. Bibcode:2014PNAS..111E2066M. doi:10.1073/pnas.1313093111. ISSN 0027-8424. PMC 4024891. PMID 24778245.
- Huang, Wenjing; Pach, Daniel; Napadow, Vitaly; Park, Kyungmo; Long, Xiangyu; Neumann, Jane; Maeda, Yumi; Nierhaus, Till; Liang, Fanrong; Witt, Claudia M.; Harrison, Ben J. (9 April 2012). "Characterizing Acupuncture Stimuli Using Brain Imaging with fMRI – A Systematic Review and Meta-Analysis of the Literature". PLOS ONE. 7 (4): e32960. Bibcode:2012PLoSO...732960H. doi:10.1371/journal.pone.0032960. PMC 3322129. PMID 22496739.
- Chae, Younbyoung; Chang, Dong-Seon; Lee, Soon-Ho; Jung, Won-Mo; Lee, In-Seon; Jackson, Stephen; Kong, Jian; Lee, Hyangsook; Park, Hi-Joon; Lee, Hyejung; Wallraven, Christian (March 2013). "Inserting Needles Into the Body: A Meta-Analysis of Brain Activity Associated With Acupuncture Needle Stimulation". The Journal of Pain. 14 (3): 215–222. doi:10.1016/j.jpain.2012.11.011. PMID 23395475.
- Fox, Kieran C. R.; Nijeboer, Savannah; Dixon, Matthew L.; Floman, James L.; Ellamil, Melissa; Rumak, Samuel P.; Sedlmeier, Peter; Christoff, Kalina (2014). "Is meditation associated with altered brain structure? A systematic review and meta-analysis of morphometric neuroimaging in meditation practitioners". Neuroscience & Biobehavioral Reviews. 43: 48–73. doi:10.1016/j.neubiorev.2014.03.016. PMID 24705269.
- Raffone, Antonino; Srinivasan, Narayanan (2010). "The exploration of meditation in the neuroscience of attention and consciousness". Cognitive Processing. 11 (1): 1–7. doi:10.1007/s10339-009-0354-z. PMID 20041276.
- Xu, J; Vik, A; Groote, IR; Lagopoulos, J; Holen, A; Ellingsen, Ø; Håberg, AK; Davanger, S (2014). "Nondirective meditation activates default mode network and areas associated with memory retrieval and emotional processing". Front. Hum. Neurosci. 8 (86): 86. doi:10.3389/fnhum.2014.00086. PMC 3935386. PMID 24616684.
- Picchioni, Dante; Duyn, Jeff H.; Horovitz, Silvina G. (2013-10-15). "Sleep and the functional connectome". NeuroImage. 80: 387–396. doi:10.1016/j.neuroimage.2013.05.067. PMC 3733088. PMID 23707592.
- McKenna, Benjamin S.; Eyler, Lisa T. (2012). "Overlapping prefrontal systems involved in cognitive and emotional processing in euthymic bipolar disorder and following sleep deprivation: A review of functional neuroimaging studies". Clinical Psychology Review. 32 (7): 650–663. doi:10.1016/j.cpr.2012.07.003. PMC 3922056. PMID 22926687.
- Basner, Mathias; Rao, Hengyi; Goel, Namni; Dinges, David F (October 2013). "Sleep deprivation and neurobehavioral dynamics". Current Opinion in Neurobiology. 23 (5): 854–863. doi:10.1016/j.conb.2013.02.008. PMC 3700596. PMID 23523374.
- Carhart-Harris, Robin L.; Erritzoe, David; Williams, Tim; Stone, James M.; Reed, Laurence J.; Colasanti, Alessandro; Tyacke, Robin J.; Leech, Robert; Malizia, Andrea L.; Murphy, Kevin; Hobden, Peter; Evans, John; Feilding, Amanda; Wise, Richard G.; Nutt, David J. (2012). "Neural correlates of the psychedelic state as determined by fMRI studies with psilocybin". PNAS. 109 (6): 2138–2143. doi:10.1073/pnas.1119598109. PMC 3277566. PMID 22308440.
- Carhart-Harris, Robin L.; Muthukumaraswamy, Suresh; Roseman, Leor; Kaelen, Mendel; Droog, Wouter; Murphy, Kevin; Tagliazucchi, Enzo; Schenberg, Eduardo E.; Nest, Timothy; Orban, Csaba; Leech, Robert; Williams, Luke T.; Williams, Tim M.; Bolstridge, Mark; Sessa, Ben; McGonigle, John; Sereno, Martin I.; Nichols, David; Hellyer, Peter J.; Hobden, Peter; Evans, John; Singh, Krish D.; Wise, Richard G.; Curran, H. Valerie; Feilding, Amanda; Nutt, David J. (26 April 2016). "Neural correlates of the LSD experience revealed by multimodal neuroimaging". Proceedings of the National Academy of Sciences. 113 (17): 4853–4858. Bibcode:2016PNAS..113.4853C. doi:10.1073/pnas.1518377113. PMC 4855588. PMID 27071089.
- Kringelbach, Morten L.; Green, Alexander L.; Aziz, Tipu Z. (2011-05-02). "Balancing the Brain: Resting State Networks and Deep Brain Stimulation". Frontiers in Integrative Neuroscience. 5: 8. doi:10.3389/fnint.2011.00008. PMC 3088866. PMID 21577250.
- Sripada, Rebecca K.; King, Anthony P.; Welsh, Robert C.; Garfinkel, Sarah N.; Wang, Xin; Sripada, Chandra S.; Liberzon, Israel (2012). "Neural Dysregulation in Posttraumatic Stress Disorder". Psychosomatic Medicine. 74 (9): 904–911. doi:10.1097/PSY.0b013e318273bf33. ISSN 0033-3174. PMC 3498527. PMID 23115342.
- Akiki, Teddy J.; Averill, Christopher L.; Abdallah, Chadi G. (2017). "A Network-Based Neurobiological Model of PTSD: Evidence From Structural and Functional Neuroimaging Studies". Current Psychiatry Reports. 19 (11): 81. doi:10.1007/s11920-017-0840-4. ISSN 1523-3812. PMC 5960989. PMID 28924828.
- Voss, Michelle W.; Soto, Carmen; Yoo, Seungwoo; Sodoma, Matthew; Vivar, Carmen; van Praag, Henriette (April 2019). "Exercise and Hippocampal Memory Systems". Trends in Cognitive Sciences. 23 (4): 318–333. doi:10.1016/j.tics.2019.01.006. PMC 6422697. PMID 30777641.
- Shao, Mengling; Lin, Huiyan; Yin, Desheng; Li, Yongjie; Wang, Yifan; Ma, Junpeng; Yin, Jianzhong; Jin, Hua (2019-10-01). Rao, Hengyi (ed.). "Learning to play badminton altered resting-state activity and functional connectivity of the cerebellar sub-regions in adults". PLOS ONE. 14 (10): e0223234. doi:10.1371/journal.pone.0223234. ISSN 1932-6203. PMC 6771995. PMID 31574108.
- Muraskin, Jordan; Dodhia, Sonam; Lieberman, Gregory; Garcia, Javier O.; Verstynen, Timothy; Vettel, Jean M.; Sherwin, Jason; Sajda, Paul (December 2016). "Brain dynamics of post-task resting state are influenced by expertise: Insights from baseball players: Brain Dynamics of Post-Task Resting State". Human Brain Mapping. 37 (12): 4454–4471. doi:10.1002/hbm.23321. PMC 5113676. PMID 27448098.
- Foster, Brett L.; Parvizi, Josef (2012-03-01). "Resting oscillations and cross-frequency coupling in the human posteromedial cortex". NeuroImage. 60 (1): 384–391. doi:10.1016/j.neuroimage.2011.12.019. ISSN 1095-9572. PMC 3596417. PMID 22227048.
- Morris, Peter G.; Smith, Stephen M.; Barnes, Gareth R.; Stephenson, Mary C.; Hale, Joanne R.; Price, Darren; Luckhoo, Henry; Woolrich, Mark; Brookes, Matthew J. (2011-10-04). "Investigating the electrophysiological basis of resting state networks using magnetoencephalography". Proceedings of the National Academy of Sciences. 108 (40): 16783–16788. Bibcode:2011PNAS..10816783B. doi:10.1073/pnas.1112685108. ISSN 0027-8424. PMC 3189080. PMID 21930901.
- Fair, D. A.; Cohen, A. L.; Dosenbach, N. U. F.; Church, J. A.; Miezin, F. M.; Barch, D. M.; Raichle, M. E.; Petersen, S. E.; Schlaggar, B. L. (2008). "The maturing architecture of the brain's default network". Proceedings of the National Academy of Sciences. 105 (10): 4028–32. Bibcode:2008PNAS..105.4028F. doi:10.1073/pnas.0800376105. PMC 2268790. PMID 18322013.
- Morcom, Alexa M.; Fletcher, Paul C. (October 2007). "Does the brain have a baseline? Why we should be resisting a rest". NeuroImage. 37 (4): 1073–1082. doi:10.1016/j.neuroimage.2006.09.013. PMID 17052921.
External links
![]() |
Wikimedia Commons has media related to Default mode network. |