Ocean heat content
In oceanography and climatology, ocean heat content (OHC) is a term for the energy absorbed by the ocean, which is stored as internal energy or enthalpy. Changes in the ocean heat content play an important role in the sea level rise, because of thermal expansion.
.png.webp)
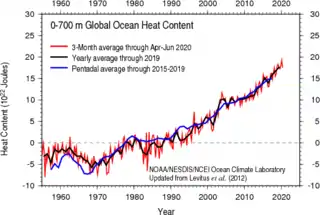
Ocean warming accounts for 90% of the energy accumulation from global warming between 1971 and 2010.[1] About one third of that extra heat has been estimated to propagate to depth below 700 meters.[2] Beyond the direct impact of thermal expansion, ocean warming contributes to an increased rate of ice melting in the fjords of Greenland [3] and Antarctic ice sheets.[4] Warmer oceans are also responsible for coral bleaching.[5]
Definition and measurement
The areal density of ocean heat content between two depth levels is defined using a definite integral:[6]
where is seawater density, is the specific heat of sea water, h2 is the lower depth, h1 is the upper depth, and is the temperature profile. In SI units, has units of J·m−2. Integrating this density over an ocean basin, or entire ocean, gives the total heat content, as indicated in the figure to right. Thus, the total heat content is the product of the density, specific heat capacity, and the volume integral of temperature over the three-dimensional region of the ocean in question.
Ocean heat content can be estimated using temperature measurements obtained by a Nansen bottle, an ARGO float, or ocean acoustic tomography. The World Ocean Database Project is the largest database for temperature profiles from all of the world’s oceans.
The upper Ocean heat content in most North Atlantic regions is dominated by heat transport convergence (a location where ocean currents meet), without large changes to temperature and salinity relation.[7]
Recent changes
Several studies in recent years have found a multi-decadal rise in OHC of the deep and upper ocean regions and attribute the heat uptake to anthropogenic warming.[8] Studies based on ARGO indicate that ocean surface winds, especially the subtropical trade winds in the Pacific Ocean, change ocean heat vertical distribution.[9] This results in changes among ocean currents, and an increase of the subtropical overturning, which is also related to the El Niño and La Niña phenomenon. Depending on stochastic natural variability fluctuations, during La Niña years around 30% more heat from the upper ocean layer is transported into the deeper ocean. Model studies indicate that ocean currents transport more heat into deeper layers during La Niña years, following changes in wind circulation.[10][11] Years with increased ocean heat uptake have been associated with negative phases of the interdecadal Pacific oscillation (IPO).[12] This is of particular interest to climate scientists who use the data to estimate the ocean heat uptake.
A study in 2015 concluded that ocean heat content increases by the Pacific Ocean, were compensated by an abrupt distribution of OHC into the Indian Ocean.[13]
See also
- Effects of climate change on oceans
- Sea surface temperature
Oceans portal
References
- IPCC AR5 WG1 (2013). "Summary for policymakers" (PDF). www.climatechange2013.org. Retrieved 15 July 2016.
- "Study: Deep Ocean Waters Trapping Vast Store of Heat". Climate Central. 2016.
- Church, J.A. (2013). "Sea Level Change". In Intergovernmental Panel On Climate Change (ed.). Sea Level Change, pp. 1137-1216. Climate Change 2013 – The Physical Science Basis: Working Group I Contribution to the Fifth Assessment Report of the Intergovernmental Panel on Climate Change. pp. 1137–1216. doi:10.1017/cbo9781107415324.026. ISBN 9781107415324. Retrieved 2019-02-05.
- Jenkins, Adrian; et al. (2016). "Decadal Ocean Forcing and Antarctic Ice Sheet Response: Lessons from the Amundsen Sea | Oceanography". tos.org. Retrieved 2019-02-05.
- "The Great Barrier Reef: a catastrophe laid bare". The Guardian. 6 June 2016.
- Dijkstra, Henk A. (2008). Dynamical oceanography ([Corr. 2nd print.] ed.). Berlin: Springer Verlag. p. 276. ISBN 9783540763758.
- Sirpa Häkkinen, Peter B Rhines, and Denise L Worthen (2015). "Heat content variability in the North Atlantic Ocean in ocean reanalyses". Geophys Res Lett. 42 (8): 2901–2909. Bibcode:2015GeoRL..42.2901H. doi:10.1002/2015GL063299. PMC 4681455. PMID 26709321.CS1 maint: uses authors parameter (link)
- Abraham; et al. (2013). "A review of global ocean temperature observations: Implications for ocean heat content estimates and climate change". Reviews of Geophysics. 51 (3): 450–483. Bibcode:2013RvGeo..51..450A. CiteSeerX 10.1.1.594.3698. doi:10.1002/rog.20022.
- Balmaseda, Trenberth & Källén (2013). "Distinctive climate signals in reanalysis of global ocean heat content". Geophysical Research Letters. 40 (9): 1754–1759. Bibcode:2013GeoRL..40.1754B. doi:10.1002/grl.50382. Essay Archived 2015-02-13 at the Wayback Machine
- Meehl; et al. (2011). "Model-based evidence of deep-ocean heat uptake during surface-temperature hiatus periods". Nature Climate Change. 1 (7): 360–364. Bibcode:2011NatCC...1..360M. doi:10.1038/nclimate1229.
- Rob Painting (2 October 2011). "The Deep Ocean Warms When Global Surface Temperatures Stall". SkepticalScience.com. Retrieved 15 July 2016.
- Rob Painting (24 June 2013). "A Looming Climate Shift: Will Ocean Heat Come Back to Haunt us?". SkepticalScience.com. Retrieved 15 July 2016.
- Sang-Ki Lee, Wonsun Park, Molly O. Baringer, Arnold L. Gordon, Bruce Huber & Yanyun Liu (18 May 2015). "Pacific origin of the abrupt increase in Indian Ocean heat content during the warming hiatus" (PDF). Nature Geoscience. 8 (6): 445–449. Bibcode:2015NatGe...8..445L. doi:10.1038/ngeo2438.CS1 maint: uses authors parameter (link)
- Cheng L. J., Zhu J. (2014). "Artifacts in variations of ocean heat content induced by the observation system changes". Geophysical Research Letters. 41 (20): 7276–7283. Bibcode:2014GeoRL..41.7276C. doi:10.1002/2014GL061881.