Mid-Pleistocene Transition
The Mid-Pleistocene Transition (MPT),[1] also known as the Mid-Pleistocene Revolution (MPR),[2] is a fundamental change in the behaviour of glacial cycles during the Quaternary glaciations. The transition happened approximately 1.25–0.7 million years ago, in the Pleistocene epoch.[3] Before the MPT, the glacial cycles were dominated by a 41,000 year periodicity with low-amplitude, thin ice sheets and a linear relationship to the Milankovitch forcing from axial tilt. After the MPT there have been strongly asymmetric cycles with long-duration cooling of the climate and build-up of thick ice sheets, followed by a fast change from extreme glacial conditions to a warm interglacial. The cycle lengths have varied, with an average length of approximately 100,000 years.[1][3]
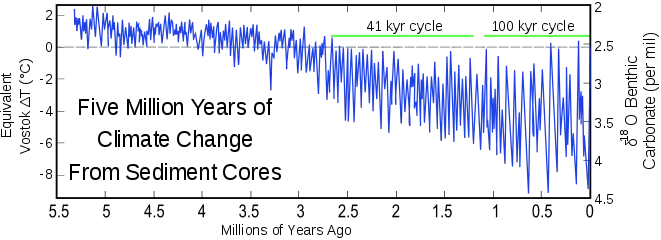
The Mid-Pleistocene Transition was long a problem to explain, as described in the article 100,000-year problem. The MPT can now be reproduced by numerical models that assume a decreasing level of atmospheric carbon dioxide, a high sensitivity to this decrease, and gradual removal of regoliths from northern hemisphere areas subject to glacial processes during the Quaternary.[1] The reduction in CO
2 may be related to changes in volcanic outgassing, burial of ocean sediments, carbonate weathering or iron fertilization of oceans from glacially induced dust.[4]
Regoliths are believed to affect glaciation because ice with its base on regolith at the pressure melting point will slide with relative ease, which limits the thickness of the ice sheet. Before the Quaternary, northern North America and northern Eurasia are believed to have been covered by thick layers of regoliths, which have been worn away over large areas by subsequent glaciations.[3] Later glaciations were increasingly based on core areas, with thick ice sheets strongly coupled to bare bedrock.[3][4][1]
However, a 2020 study concluded that ice age terminations might have been influenced by obliquity since the Mid-Pleistocene Transition, which caused stronger summers in the Northern Hemisphere.[5]
A study published in January 2021 [6] approaches the MPT in a new way based on a revisited Milankovitch theory in which the solar and orbital forcing of the climate system occurs under the mediation of very long-period Rossby waves winding around the subtropical gyres. Due to their specific properties, the so-called Gyral Rossby Waves (GRWs) are resonantly forced in subharmonic modes. This means that the forcing efficiency strongly depends on the deviation between the forcing period and the closest natural period of GRWs among the different subharmonic modes.[6]
Before the MPT, GRWs were resonantly forced from variations in obliquity whose period is 41 Ka: forcing resulting from variations in obliquity is much higher than that resulting from variations in eccentricity, whose period is nearly 100 Ka. But the natural period closest to 41 Ka is 49.2 Ka related to the subharmonic mode n10.[6] This implies that the radius of the subtropical gyres was 20% smaller than it is nowadays so that the resonance period and the forcing period are fine-tuned. This has been observed by considering together paleothermometers at two sites in the Tasman Sea.[7]
Nearly 1.2 Ma ago, the forcing period of eccentricity became remarkably close to the natural period of GRWs related to the subharmonic mode n11, namely 98.3 Ka, so that a competition occurred between obliquity and eccentricity forcing. The fine tuning of the forcing period related to eccentricity with one of the natural periods of GRWs modified the dominant resonance period which became that of the eccentricity.
A transition comparable to the MPT occurred at the hinge of the Pliocene and the Pleistocene, the periods being 10 times higher than during the MPT.[6]
See also
References
- Brovkin, V.; Calov, R.; Ganopolski, A.; Willeit, M. (April 2019). "Mid-Pleistocene transition in glacial cycles explained by declining CO2 and regolith removal | Science Advances". Science Advances. 5 (4): eaav7337. doi:10.1126/sciadv.aav7337. PMC 6447376. PMID 30949580.
- Mark A. Maslin and Andy J. Ridgwell (2005): Mid-Pleistocene revolution and the ‘eccentricity myth’, Geological Society, London, Special Publications, 247, 19-34, 1 January 2005
- Clark, Peter U; Archer, David; Pollard, David; Blum, Joel D; Rial, Jose A; Brovkin, Victor; Mix, Alan C; Pisias, Nicklas G; Roy, Martin (2006). "The middle Pleistocene transition: characteristics, mechanisms, and implications for long-term changes in atmospheric pCO2" (PDF). Quaternary Science Reviews. Elsevier. 25 (23–24): 3150–3184. Bibcode:2006QSRv...25.3150C. doi:10.1016/j.quascirev.2006.07.008.
- Chalk et al. (2017): Causes of ice age intensification across the Mid-Pleistocene Transition, PNAS December 12, 2017 114 (50) 13114-13119
- Petra Bajo; et al. (2020). "Persistent influence of obliquity on ice age terminations since the Middle Pleistocene transition". 367 (6483). Science. pp. 1235–1239. doi:10.1126/science.aaw1114.
- Pinault, Jean-Louis (January 2021). "Resonantly Forced Baroclinic Waves in the Oceans: A New Approach to Climate Variability". Journal of Marine Science and Engineering. 9 (1): 13. doi:10.3390/jmse9010013.
- McClymont, E. L.; Elmore, A. C.; Kender, S.; Leng, M. J.; Greaves, M.; Elderfield, H. (2016-06-30). "Pliocene-Pleistocene evolution of sea surface and intermediate water temperatures from the southwest Pacific". Paleoceanography. 31 (6): 895–913. doi:10.1002/2016PA002954. ISSN 0883-8305.