CLARITY
CLARITY [1] is a method of making brain tissue transparent using acrylamide-based hydrogels built from within, and linked to, the tissue, and as defined in the initial paper, represents "transformation of intact biological tissue into a hybrid form in which specific components are replaced with exogenous elements that provide new accessibility or functionality".[1] When accompanied with antibody or gene-based labeling, CLARITY enables highly detailed pictures of the protein and nucleic acid structure of organs, especially the brain. It was developed by Kwanghun Chung and Karl Deisseroth at the Stanford University School of Medicine.[2]
Subsequent published papers have applied the CLARITY method of building acrylamide-based tissue-gel hybrids within tissue for improved optical and molecular access to Alzheimer's disease human brains,[3] mouse spinal cords,[4] multiple sclerosis animal models,[5] and plants.[6] CLARITY has also been combined with other technologies to develop new microscopy methods including confocal expansion microscopy and CLARITY-optimized light sheet microscopy (COLM).[7]
Procedure
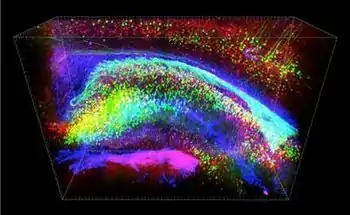
The process of applying CLARITY imaging begins with a postmortem tissue sample. Next a series of chemical treatments must be applied to achieve transparency, in which the lipid content of the sample is removed, while almost all of the original proteins and nucleic acids are left in place.[1] The purpose of this is to make the tissue transparent and thus amenable to detailed microscopic investigation of its constituent functional parts (which are predominantly proteins and nucleic acids). To accomplish this, the preexisting protein structure has to be placed in a transparent scaffolding which preserves it, while the lipid components are removed. This 'scaffolding' is made up of hydrogel monomers such as acrylamide. The addition of molecules like formaldehyde, paraformaldehyde, or glutaraldehyde can facilitate attachment of the scaffolding to the proteins and nucleic acids that are to be preserved, and the addition of heat is necessary to establish the actual linkages between the cellular components and the acrylamide.[8]
Once this step is complete, the protein and nucleic acid components of the target tissue's cells are held firmly in place, while the lipid components remain detached. Lipids are then removed over 1–2 weeks of passive diffusion in detergent, or accelerated by electrophoretic methods to only hours to days.[9][10] As they pass through, the detergent's lipophilic properties enable it to pick up and excise any lipids encountered along the way. Lipophilic dyes as DiI are removed, however there are CLARITY-compatible lipophilic dyes that can be fixated to neighbouring proteins.[11] The large majority of non-lipid molecules, such as proteins and DNA, remain unaffected by this procedure, thanks to the acrylamide gel and chemical properties of the molecules involved.[8]
As reported in the initial paper, the tissue expands during this process, but as needed can be restored to its initial dimensions with a final step of incubation in refractive index matching solution.[1]
By this stage in the process, the sample has been fully prepared for imaging. The contrast for imaging can come from endogenous fluorescent molecules, from nucleic acid (DNA or RNA) labels, or from immunostaining, whereby antibodies that bind specifically to a certain target substance are used. In addition, these antibodies are labeled with Fluorescent tags that are the key to final imaging result. Standard confocal, two-photon, or light-sheet imaging methods are all suitable to then detect the fluorescence emitted down to the scale of protein localization, thus resulting in the final highly detailed and three-dimensional images that CLARITY produces.[8]
After a sample has been immunostained for an image, it is possible to remove the antibodies and re-apply new ones, thus enabling a sample to be imaged multiple times and targeting multiple protein types.[12][13]
Applications
In terms of brain imaging, the ability for CLARITY imaging to reveal specific structures in such unobstructed detail has led to promising avenues of future applications including local circuit wiring (especially as it relates to the Connectome Project), relationships between neural cells, roles of subcellular structures, better understanding of protein complexes, and imaging of nucleic acids and neurotransmitters.[1] An example of a discovery made through CLARITY imaging is a peculiar 'ladder' pattern where neurons connected back to themselves and their neighbors, which has been observed in animals to be connected to autism-like behaviors.[14]
CLARITY can be used with little or no modifications to clear most other organs such as liver, pancreas, spleen, testis, and ovaries and other species such as zebrafish. While bone requires a simple decalcification step, similarly, plant tissue requires an enzymatic degradation of the cell wall.[9]
NIH director Francis Collins has already expressed his hopes for this emergent technology, saying:[15]
"CLARITY is powerful. It will enable researchers to study neurological diseases and disorders, focusing on diseased or damaged structures without losing a global perspective. That’s something we’ve never before been able to do in three dimensions."
Limitations
Although the CLARITY procedure has attained unprecedented levels of protein retention after lipid extraction, the technique still loses an estimated 8% of proteins per instance of detergent electrophoresis.[12] Repeated imaging of a single sample would only amplify this loss, as antibody removal is commonly accomplished via the same detergent process that creates the original sample.[8]
Other disadvantages of the technique are the length of time it takes to create and image a sample (the immunohistochemical staining alone takes up to six weeks to perform), and the fact that the acrylamide used is highly toxic and carcinogenic.
See also
References
- Chung K, Wallace J, Kim SY, Kalyanasundaram S, Andalman AS, Davidson TJ, Mirzabekov JJ, Zalocusky KA, Mattis J, Denisin AK, Pak S, Bernstein H, Ramakrishnan C, Grosenick L, Gradinaru V, Deisseroth K (May 2013). "Structural and molecular interrogation of intact biological systems". Nature. 497 (7449): 332–7. doi:10.1038/nature12107. PMC 4092167. PMID 23575631.
- Underwood E (April 2013). "Neuroscience. Tissue imaging method makes everything clear". Science. 340 (6129): 131–2. doi:10.1126/science.340.6129.131. PMID 23580500.
- Ando K, Laborde Q, Lazar A, Godefroy D, Youssef I, Amar M, Pooler A, Potier MC, Delatour B, Duyckaerts C (September 2014). "Inside Alzheimer brain with CLARITY: senile plaques, neurofibrillary tangles and axons in 3-D". Acta Neuropathologica. 128 (3): 457–9. doi:10.1007/s00401-014-1322-y. PMC 4131133. PMID 25069432.
- Zhang MD, Tortoriello G, Hsueh B, Tomer R, Ye L, Mitsios N, Borgius L, Grant G, Kiehn O, Watanabe M, Uhlén M, Mulder J, Deisseroth K, Harkany T, Hökfelt TG (March 2014). "Neuronal calcium-binding proteins 1/2 localize to dorsal root ganglia and excitatory spinal neurons and are regulated by nerve injury". Proceedings of the National Academy of Sciences of the United States of America. 111 (12): E1149-58. doi:10.1073/pnas.1402318111. PMC 3970515. PMID 24616509.
- Spence RD, Kurth F, Itoh N, Mongerson CR, Wailes SH, Peng MS, MacKenzie-Graham AJ (November 2014). "Bringing CLARITY to gray matter atrophy". NeuroImage. 101: 625–32. doi:10.1016/j.neuroimage.2014.07.017. PMC 4437539. PMID 25038439.
- Palmer WM, Martin AP, Flynn JR, Reed SL, White RG, Furbank RT, Grof CP (September 2015). "PEA-CLARITY: 3D molecular imaging of whole plant organs". Scientific Reports. 5: 13492. doi:10.1038/srep13492. PMC 4556961. PMID 26328508.
- Tomer R, Ye L, Hsueh B, Deisseroth K (July 2014). "Advanced CLARITY for rapid and high-resolution imaging of intact tissues". Nature Protocols. 9 (7): 1682–97. doi:10.1038/nprot.2014.123. PMC 4096681. PMID 24945384.
- Geaghan-Breiner C (2013). "CLARITY Brain Imaging". Stanford University.
- Jensen KH, Berg RW (December 2017). "Advances and perspectives in tissue clearing using CLARITY". Journal of Chemical Neuroanatomy. 86: 19–34. doi:10.1016/j.jchemneu.2017.07.005. PMID 28728966. S2CID 27575056.
- Lee E, Choi J, Jo Y, Kim JY, Jang YJ, Lee HM, Kim SY, Lee HJ, Cho K, Jung N, Hur EM, Jeong SJ, Moon C, Choe Y, Rhyu IJ, Kim H, Sun W (January 2016). "ACT-PRESTO: Rapid and consistent tissue clearing and labeling method for 3-dimensional (3D) imaging". Scientific Reports. 6 (1): 18631. doi:10.1038/srep18631. PMC 4707495. PMID 26750588.
- Jensen KH, Berg RW (September 2016). "CLARITY-compatible lipophilic dyes for electrode marking and neuronal tracing". Scientific Reports. 6: 32674. doi:10.1038/srep32674. PMC 5011694. PMID 27597115.
- Shen, Helen (April 10, 2013). "See-through brains clarify connections". Nature News.
- Murray E, Cho JH, Goodwin D, Ku T, Swaney J, Kim SY, Choi H, Park YG, Park JY, Hubbert A, McCue M, Vassallo S, Bakh N, Frosch MP, Wedeen VJ, Seung HS, Chung K (December 2015). "Simple, Scalable Proteomic Imaging for High-Dimensional Profiling of Intact Systems". Cell. 163 (6): 1500–14. doi:10.1016/j.cell.2015.11.025. PMC 5275966. PMID 26638076.
- "See-through brains". Nature Video.
- Collins F. "The Brain: Now You See It, Soon You Won't". NIH Directors Blog. NIH.